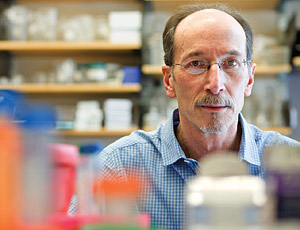
David M. Warshaw, Ph.D. Professor and Chair of Molecular Physiology & Biophysics
Office: HSRF 116
Phone: 802-656-4300
Email: David.Warshaw@med.uvm.edu
Myosin Molecular Motors
I received my B.S. in Electrical Engineering from Rutgers University and Ph.D. in Physiology & Biophysics from the University of Vermont. As a postdoc with Fredric Fay at UMass Medical, I studied single smooth muscle cell mechanics. My present research focuses on the structure and function of cardiac muscle contractile proteins as well as non-muscle molecular motors using single molecule biophysical techniques such as laser trapping and super-resolution microscopy. Presently, my lab has two research foci. One area focuses on the molecular mechanism by which myosin binding protein-C modulates cardiac and skeletal muscle contractility, using an in vitro muscle model systems. The other focus is in vitro 3D model systems of intracellular cargo transport by myosin motors. I have been the Principal Investigator of a National Institutes of Health (NIH) Program Project Grant focused on the molecular basis of genetic heart failure. I am an Established Investigator and Fellow of the American Heart Association and a Fellow of the Biophysical Society. I have organized numerous International Conferences and Symposia, including the Gordon Conference on “Muscle Contractile Proteins” (1999, 2002) and was the program co-chair of the 2009 Biophysical Society annual meeting. I have and continue to serve on numerous NIH review panels and was a member of the Scientific Advisory Panel for the NIH Nanomedicine Initiative. I have trained 26 pre- and postdoctoral fellows of which 17 have gone on to university faculty positions.
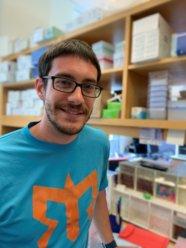
Brandon M. Bensel, Ph.D.
Postdoctoral Fellow
Office: HSRF115 Phone: 802-656-3841
Email: Brandon.bensel@uvm.edu
Myosin molecular motors
The proper delivery of cellular cargoes via intracellular transport is necessary to ensure healthy cellular function. To date, much work has been done to understand the mechanisms which regulate cargo transport in vitro, yet much of this work is done in 2 dimensions with a single type of motor on a rigid cargo, which may not recapitulate the physiological system. My work aims to understand how teams of multiple kinesin and myosin molecular motors navigate complex 3-dimensional networks of microtubules and actin filaments while bound to artificial liposome cargoes.
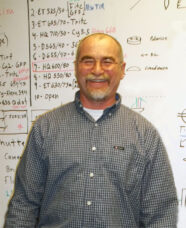
Guy Kennedy
Research Engineer
Office: HSRF 115
Phone: 802-656-9420
Email: guy.kennedy@uvm.edu
My goal is to design, develop, fabricate and support world class microscopy systems for research in molecular physiology. Our research demands high sensitivity with fast temporal and precise spatial resolution. These systems include integrated Laser Optical Tweezers with dual color Total Internal Reflection Microscopy (TIRFM) along with single molecule detection, manipulation and tracking capabilities. Mechanical measurements of pico-newton force, nanometer displacement, and pico-newtons/ nanometer stiffness are used to address questions in single molecules. These techniques are applied to protein ensembles, intercellular dynamics, and molecular protein- protein interactions. High speed fluorescent imaging of single molecule dynamics are possible using state of the art ICCD cameras with TIR and far field illumination. Recent initiatives include high speed 3D tracking, STORM, and PALM Super Resolution microscopy. Myosin, Kinesin, C-Protein, Actin, Microtubules and other Cytoskeletal proteins are studied with our techniques.Guy Kennedy Research Engineer Office: HSRF 115 Phone: 802-656-9420 Email: guy.kennedy@uvm.edu My goal is to design, develop, fabricate and support world class microscopy systems for research in molecular physiology. Our research demands high sensitivity with fast temporal and precise spatial resolution. These systems include integrated Laser Optical Tweezers with dual color Total Internal Reflection Microscopy (TIRFM) along with single molecule detection, manipulation and tracking capabilities. Mechanical measurements of pico-newton force, nanometer displacement, and pico-newtons/ nanometer stiffness are used to address questions in single molecules. These techniques are applied to protein ensembles, intercellular dynamics, and molecular protein- protein interactions. High speed fluorescent imaging of single molecule dynamics are possible using state of the art ICCD cameras with TIR and far field illumination. Recent initiatives include high speed 3D tracking, STORM, and PALM Super Resolution microscopy. Myosin, Kinesin, C-Protein, Actin, Microtubules and other Cytoskeletal proteins are studied with our techniques.
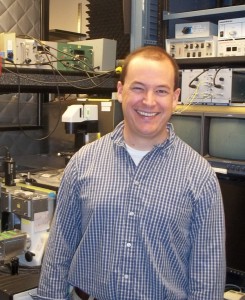
Shane Nelson, Ph.D.
Faculty Scientist
Office: HSRF 115
Phone: 802-656-3820
Email: Shane.Nelson@uvm.edu
Myosin V Vesicle Transport in COS-7 cells My work focuses on Myosin Va (myoVa), which is an intracellular cargo transporter. Based on in vitro experiments, a single myoVa molecule could perform this function as it can move processively along actin tracks in a “hand-over-hand” fashion. However, this has yet to be demonstrated in vivo. The cellular context presents numerous challenges to myoVa processivity such as the dense cytoskeletal network, actin-binding proteins, and other motors that share cargo transport duties with myoVa. To study the in vivo motion and processivity of myoVa, I introduced quantum dot (Qdot) labeled myoVa molecules into cultured fibroblast (COS-7) cells by pinocytosis, and observed the motion of individual motor molecules by TIRF microscopy. I have shown that individual myoVa molecules undergo a random walk by making frequent turns onto intersecting actin filaments in the densely packed subplasmalemmal actin cortex. My current efforts are to begin to understand how myoVa is targeted to its cargo and how multiple motor molecules coordinate their activity to bring about effective cargo transport.
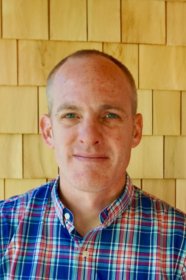
Andrew Mead, Ph.D.
Faculty Scientist
Lab: HSRF115
Phone: 802-656-3820
Muscle powers a diverse range of biological functions in vertebrates, from the rapid vocalizations of songbirds to the slow swimming of carp. Remarkably, muscle’s basic force-generating structure, the sarcomere, is highly conserved among vertebrate muscles, as are the genes that encode its constituent proteins. I use the swimming muscles of zebrafish larvae as a model system to ask basic questions about how sarcomeres produce force and motion, and in particular how their mechanical characteristics can be ‘tuned’. Larval muscles develop and begin to function within a few days of fertilization, meaning that I can generate experimental muscles, with genetic modifications or other interventions, comparatively easily and rapidly. The orientation of muscle fibers within the larval tail allows me to apply suite of classical muscle mechanics experiments to quantify the effects of these interventions on muscle force, shortening velocity, and power. My current work focuses on Myosin Binding Protein C (MyBP-C), which is understood to be an important regulator of cardiac and skeletal muscle function. Single molecule biophysical studies by the Warshaw group and others have begun to uncover the molecular mechanisms by which the various isoforms of MyBP-C may affect muscle mechanics. By expressing these isoforms in zebrafish we can observe how these molecular insights play out in the higher order setting of an intact, living muscle.
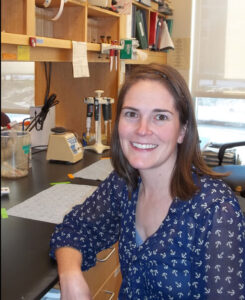
Samantha Previs
Research Technician
Office: HSRF 115
Phone: 802-656-3841
Email: samantha.beck@uvm.edu
I am involved in experiments looking at the function of myosin-binding protein C and its role in cardiac muscle contraction, insulin granule secretion, and myosin transport on actin networks. I also provide general laboratory support by preparing reagents and ordering needed supplies.